Abstract
Large, distributed collections of miniaturized, wireless electronic devices1,2 may form the basis of future systems for environmental monitoring3, population surveillance4, disease management5 and other applications that demand coverage over expansive spatial scales. Aerial schemes to distribute the components for such networks are required, and—inspired by wind-dispersed seeds6—we examined passive structures designed for controlled, unpowered flight across natural environments or city settings. Techniques in mechanically guided assembly of three-dimensional (3D) mesostructures7,8,9 provide access to miniature, 3D fliers optimized for such purposes, in processes that align with the most sophisticated production techniques for electronic, optoelectronic, microfluidic and microelectromechanical technologies. Here we demonstrate a range of 3D macro-, meso- and microscale fliers produced in this manner, including those that incorporate active electronic and colorimetric payloads. Analytical, computational and experimental studies of the aerodynamics of high-performance structures of this type establish a set of fundamental considerations in bio-inspired design, with a focus on 3D fliers that exhibit controlled rotational kinematics and low terminal velocities. An approach that represents these complex 3D structures as discrete numbers of blades captures the essential physics in simple, analytical scaling forms, validated by computational and experimental results. Battery-free, wireless devices and colorimetric sensors for environmental measurements provide simple examples of a wide spectrum of applications of these unusual concepts.
Access options
Subscribe to Journal
Get full journal access for 1 year
199,00 €
only 3,90 € per issue
Tax calculation will be finalised during checkout.
Rent or Buy article
Get time limited or full article access on ReadCube.
from$8.99
All prices are NET prices.
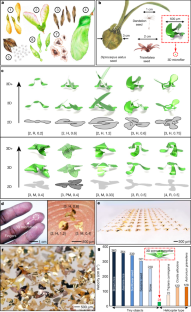
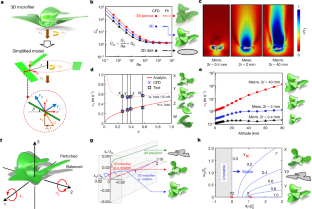
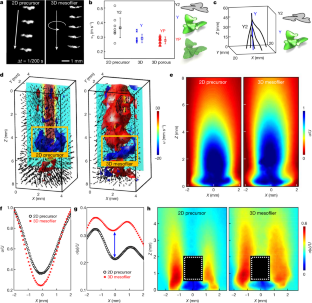
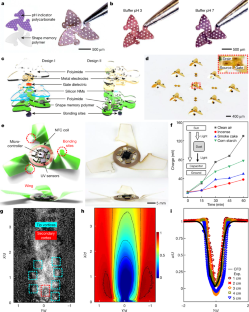
Data availability
The data that support the findings of this study are available from the corresponding author on reasonable request.
References
- 1.
Chung, H. U. et al. Binodal, wireless epidermal electronic systems with in-sensor analytics for neonatal intensive care. Science 363, eaau0780 (2019).
- 2.
Kim, B. H. et al. Mechanically guided post-assembly of 3D electronic systems. Adv. Funct. Mater. 28, 1803149 (2018).
- 3.
Jin, J., Wang, Y., Jiang, H. & Chen, X. Evaluation of microclimatic detection by a wireless sensor network in forest ecosystems. Sci. Rep. 8, 16433 (2018).
- 4.
González-Alcaide, G., Llorente, P. & Ramos-Rincón, J. M. Systematic analysis of the scientific literature on population surveillance. Heliyon 6, E05141 (2020).
- 5.
Groseclose, S. L. & Buckeridge, D. L. Public health surveillance systems: recent advances in their use and evaluation. Annu. Rev. Public Health 38, 57–79 (2017).
- 6.
Augspurger, C. K. Morphology and dispersal potential of wind-dispersed diaspores of neotropical trees. Am. J. Bot. 73, 353–363 (1986).
- 7.
Won, S. M. et al. Multimodal sensing with a three-dimensional piezoresistive structure. ACS Nano 13, 10972–10979 (2019).
- 8.
Kim, B. H. et al. Three-dimensional silicon electronic systems fabricated by compressive buckling process. ACS Nano 12, 4164–4171 (2018).
- 9.
Park, Y. et al. Three-dimensional, multifunctional neural interfaces for cortical spheroids and engineered assembloids. Sci. Adv. 7, eabf9153 (2021).
- 10.
Nathan, R. et al. Mechanisms of long-distance dispersal of seeds by wind. Nature 418, 409–413 (2002).
- 11.
Nathan, R. Long-distance dispersal of plants. Science 313, 786–788 (2006).
- 12.
Seale, M. & Nakayama, N. From passive to informed: mechanical mechanisms of seed dispersal. New Phytol. 225, 653–658 (2020).
- 13.
Cummins, C. et al. A separated vortex ring underlies the flight of the dandelion. Nature 562, 414–418 (2018).
- 14.
Rabault, J., Fauli, R. A. & Carlson, A. Curving to fly: synthetic adaptation unveils optimal flight performance of whirling fruits. Phys. Rev. Lett. 122, 024501 (2019).
- 15.
Fauli, R. A., Rabault, J. & Carlson, A. Effect of wing fold angles on the terminal descent velocity of double-winged autorotating seeds, fruits, and other diaspores. Phys. Rev. E 100, 013108 (2019).
- 16.
Xu, S. et al. Assembly of micro/nanomaterials into complex, three-dimensional architectures by compressive buckling. Science 347, 154–159 (2015).
- 17.
Zhang, Y. et al. A mechanically driven form of kirigami as a route to 3D mesostructures in micro/nanomembranes. Proc. Natl Acad. Sci. USA 112, 11757–11764 (2015).
- 18.
Zhang, Y. et al. Printing, folding and assembly methods for forming 3D mesostructures in advanced materials. Nat. Rev. Mater. 2, 17019 (2017).
- 19.
Pang, W. et al. Electro-mechanically controlled assembly of reconfigurable 3D mesostructures and electronic devices based on dielectric elastomer platforms. Natl Sci. Rev. 7, 342–354 (2020).
- 20.
Wang, X. et al. Freestanding 3D mesostructures, functional devices, and shape-programmable systems based on mechanically induced assembly with shape memory polymers. Adv. Mater. 31, 1805615 (2019).
- 21.
Greene, D. F. & Johnson, E. A. Seed mass and dispersal capacity in wind-dispersed diaspores. Oikos 67, 69–74 (1993).
- 22.
Zhang, L., Wang, X., Moran, M. D. & Feng, J. Review and uncertainty assessment of size-resolved scavenging coefficient formulations for below-cloud snow scavenging of atmospheric aerosols. Atmos. Chem. Phys. 13, 10005–10025 (2013).
- 23.
Hölzer, A. & Sommerfeld, M. New simple correlation formula for the drag coefficient of non-spherical particles. Powder Technol. 184, 361–365 (2008).
- 24.
Belmonte, A., Eisenberg, H. & Moses, E. From flutter to tumble: inertial drag and Froude similarity in falling paper. Phys. Rev. Lett. 81, 345–348 (1998).
- 25.
Zhong, H. et al. Experimental investigation of freely falling thin disks. Part 1. The flow structures and Reynolds number effects on the zigzag motion. J. Fluid Mech. 716, 228–250 (2013).
- 26.
Kim, J. T., Jin, Y., Shen, S., Dash, A. & Chamorro, L. P. Free fall of homogeneous and heterogeneous cones. Phys. Rev. Fluids 5, 093801 (2020).
- 27.
Chigurupati, N. et al. Evaluation of red cabbage dye as a potential natural color for pharmaceutical use. Int. J. Pharm. 241, 293–299 (2002).
- 28.
Binnig, J., Meyer, J. & Kasper, G. Calibration of an optical particle counter to provide PM2.5 mass for well-defined particle materials. J. Aerosol Sci. 38, 325–332 (2007).
- 29.
Shao, W., Zhang, H. & Zhou, H. Fine particle sensor based on multi-angle light scattering and data fusion. Sensors 17, 1033 (2017).
- 30.
Heo, S. Y. et al. Wireless, battery-free, flexible, miniaturized dosimeters monitor exposure to solar radiation and to light for phototherapy. Sci. Transl. Med. 10, eaau1643 (2018).
- 31.
Kang, S. K., Koo, J., Lee, Y. K. & Rogers, J. A. Advanced materials and devices for bioresorbable electronics. Acc. Chem. Res. 51, 988–998 (2018).
- 32.
Lee, G., Choi, Y. S., Yoon, H. J. & Rogers, J. A. Advances in physicochemically stimuli-responsive materials for on-demand transient electronic systems. Matter 3, 1031–1052 (2020).
- 33.
Kim, J. T., Nam, J., Shen, S., Lee, C. & Chamorro, L. P. On the dynamics of air bubbles in Rayleigh-Bénard convection. J. Fluid Mech. 891, A7 (2020).
- 34.
Kim, J. T. & Chamorro, L. P. Lagrangian description of the unsteady flow induced by a single pulse of a jellyfish. Phys. Rev. Fluids 4, 064605 (2019).
Acknowledgements
This work was supported by the Querrey Simpson Institute for Bioelectronics at Northwestern University. B.H.K. acknowledges support from the following: National Research Foundation of Korea (NRF) grants funded by the Korean government (MSIT) (nos 2019R1G1A1100737, 2020R1C1C1014980); the Nanomaterial Technology Development Program (NRF-2016M3A7B4905613) through the National Research Foundation of Korea (NRF) funded by the Ministry of Science, ICT and Future Planning; the Project for Collabo R&D between Industry, Academy and Research Institute funded by Korean Ministry of SMEs and Startups in 2020/2021 (project no. S2890749/S3104531); the Nano·Material Technology Development Program through the National Research Foundation of Korea (NRF) funded by the Ministry of Science, ICT and Future Planning (2009-0082580); the National Research Facilities and Equipment Center at the Ministry of Science and ICT (Support Program for Equipment Transfer, grant no. 1711116699); and the Glint Materials Company. K.L. acknowledges support from the State Key Laboratory of Digital Manufacturing Equipment and Technology, Huazhong University of Science and Technology (grant no. DMETKF2021010). Y.P. acknowledges support from the German Research Foundation (PA 3154/1-1). Y.Z. acknowledges support from the National Natural Science Foundation of China (grant no. 12050004), the Institute for Guo Qiang, Tsinghua University (grant no. 2019GQG1012), and the Tsinghua National Laboratory for Information Science and Technology. H.L. acknowledges support from the Creative Materials Discovery Program through the National Research Foundation of Korea (NRF) funded by the Ministry of Science and ICT (NRF-2018M3D1A1058972). S.M.W. acknowledges support from the National Research Foundation of Korea funded by the Ministry of Science and ICT of Korea (NRF-2021M3H4A1A01079367), and by the Nano Material Technology Development Program (2020M3H4A1A03084600) funded by the Ministry of Science and ICT of Korea. Y.H.J. acknowledges support from the research fund of Hanyang University (HY-202100000000832). C.H.L. acknowledges funding support from the National Science Foundation (2032529-CBET). Z.X. acknowledges support from the National Natural Science Foundation of China (grant no. 12072057), the LiaoNing Revitalization Talents Program (grant no. XLYC2007196), and Fundamental Research Funds for the Central Universities (grant no. DUT20RC(3)032). R.A. acknowledges support from the National Science Foundation Graduate Research Fellowship (NSF grant number 1842165) and a Ford Foundation Predoctoral Fellowship. We thank Jaeeun Koo for artwork in Fig. 1a.
Author information
Affiliations
Contributions
B.H.K., K.L., J.-T.K. and Y.P. contributed equally to this work. Y.H., L.P.C., Y.Z. and J.A.R. conceived the ideas and supervised the project. B.H.K., K.L., J.-T.K., Y.P., Y.H., L.P.C., Y.Z. and J.A.R. wrote the manuscript. H.J., X.W., S.M.W., H.-J.Y., G.L., W.J.J., K.H.L., Y.H.J., S.Y.H., Y.L., J.K., Y.K., Y.Y. and X.Y. performed microelectromechanical experiments. J.-T.K., T.C. and P.P. performed fluid dynamics experiments. Z.X., T.S.C., R.A., H.L., H.S., F.Z., Y.Z. and L.C. performed mechanical and electromagnetic modelling and simulation. S.H.H., J.K., S.J.O., H.L. and C.H.L. provided scientific and experimental advice. All authors commented on the manuscript.
Corresponding authors
Ethics declarations
Competing interests
The authors declare no competing interests.
Additional information
Peer review information Nature thanks Elizabeth Helbling and the other, anonymous, reviewer(s) for their contribution to the peer review of this work. Peer reviewer reports are available.
Publisher’s note Springer Nature remains neutral with regard to jurisdictional claims in published maps and institutional affiliations.
Extended data figures and tables
Extended Data Fig. 1 Bio-inspired 3D micro- and mesofliers.
Photographs of a 10 × 10 array of 3D micro- and mesofliers.
Extended Data Fig. 2 Schematic diagram of the configuration for computational fluid dynamics simulation.
(a) 3D rotational falling fliers and (b) 2D aerofoil.
Extended Data Fig. 3 Comparison of G0 and G1 across all classes of fliers.
CFD simulation results for the components G0 and G1 of the drag coefficient CD, for fliers of types R, H, M and PM, with the 2D disk as a comparison.
Extended Data Fig. 4 3D microflier with porous design.
(a) Inspiration of porosity from nature: optical images of dandelion seeds and a feather. (b) FE simulated configuration of a 3D void-free microflier (p = 0) and a 3D microflier of porosity design (p = 0.26). (c) Images of scanned thickness of a 2D precursor for a porous microflier, with top view and perspective view, respectively. (d) G0(b) and (e) G1(b) versus the attack angle for various porosities. Normalized (f) G0(b) and (g) G1(b) over their void-free values versus porosity, with the CFD values of various \(\alpha \in [0^\circ ,90^\circ ]\) and analytic fittings.
Extended Data Fig. 5 Mechanical simulation of a 3D microflier [3, H, 0.75].
Schematic images of (a) a parachute design where the blades have no rotational tilting, and (b) a rotating flier design with rotationally tilted blades. (c) Comparison of G0 and G1 between the parachute mode and rotational falling mode.
Extended Data Fig. 6 Experimental setups.
(a) Schematic (top) and photograph (bottom) of 3D-PTV experiment on free-falling mesofliers and (b) schematic (top) and photograph (bottom) of high speed PIV experiment on fixed 3D IoT fliers above a wind tunnel.
Extended Data Fig. 7 Changes in colour of a pH-responsive 3D mesoflier.
(a) Photographs of pH-responsive 3D mesoflier immersed in different buffer solutions with pH ranging from 3 to 11. (b) Response time of pH indicators after immersion into buffer solutions at different pH values.
Extended Data Fig. 8 The electrical characteristics of Si NM n-channel transistor (channel width/length = 80/20 μm) and diode integrated with 3D mesofliers.
(a) Drain current as a function of source/drain voltage for the gate voltages from 0 to 3 V. (b) The log scale transfer curves as a function of gate voltage from −7 to 7 V. (c) Current-voltage characteristics of a diode.
Extended Data Fig. 9 Experiments for particulate matter (PM).
(a) A dust generation chamber operated with kitchen blenders. Scanning electron microscope (SEM) images of fine dust generated by (b) corn starches, (c) incenses and (d) smoke cakes.
Extended Data Fig. 10 Electromagnetic performance of coils for wireless power transmission.
(a) Normalized magnetic field generated by the commercial transmission antenna with dimensions (318 mm × 338 mm × 30 mm). (b) Magnetic field strength along the line (0,0,Z) as a function of the distance Z normal to the transmission antenna for different input power Pin (1, 4, and 8 W). (c) Scattering parameters for the electromagnetic energy transfer between the coils when the NFC coil is located at the centre of the transmission antenna (0,0,0). (d) Simulated power in the NFC coil at different distance Z normal to the primary antenna with Pin = 8 W.
Supplementary information
Supplementary Information
This file includes 4 Supplementary Notes, 31 Supplementary Figures and 3 Supplementary Tables.
Supplementary Video 1
Free fall of a Tristellateia seed.
Supplementary Video 2
PIV experiment on a macroflier.
Supplementary Video 3
A 2D precursor and a 3D mesoflier above the vertical wind tunnel.
Supplementary Video 4
Free fall of a 2D precursor and a 3D microflier.
Supplementary Video 5
Free fall of micro-, meso- and macrofliers.
Supplementary Video 6
3D flow fields induced by a 2D precursor and a 3D mesoflier.
Supplementary Video 7
Instantaneous flow fields induced by a 3D electronic flier.
Rights and permissions
About this article
Cite this article
Kim, B.H., Li, K., Kim, JT. et al. Three-dimensional electronic microfliers inspired by wind-dispersed seeds. Nature 597, 503–510 (2021). https://ift.tt/3hXPTdU
-
Received:
-
Accepted:
-
Published:
-
Issue Date:
Comments
By submitting a comment you agree to abide by our Terms and Community Guidelines. If you find something abusive or that does not comply with our terms or guidelines please flag it as inappropriate.
"Electronic" - Google News
September 22, 2021 at 10:11PM
https://ift.tt/39sgyuD
Three-dimensional electronic microfliers inspired by wind-dispersed seeds - Nature.com
"Electronic" - Google News
https://ift.tt/3dmroCo
https://ift.tt/3bbj3jq
No comments:
Post a Comment